There is an increasing demand for biosensors in the food industry. In the past, little attention was given to using biosensors to examine food for pathogens. However, with a rise of incidents involving contaminated food there is now a need for a sensor that can accurately and quickly determine if food is contaminated, explains Rabi Ranjan Naik.
A Biosensor is an analytical tool, used for the detection of an analyte that combines a biological component with a physio-chemical detector. Biosensors are an important option in the agricultural and food sectors to control production processes and ensure food quality and safety by reliable, fast and cost-effective procedures. Biosensors are promising alternatives to conventional analytical tools since they offer advantages in size, cost, specificity, rapid response, precision, and sensitivity.
According to the World Health Organization (WHO), each year millions of people around the world are affected by foodborne diseases. Biological risks produced by bacteria, viruses and biotoxins, chemical substances (e.g., additives), food nutrients used to add product value, pesticides, veterinary drugs residues, and processing operations and technologies that may generate polycyclic aromatic hydrocarbons (PAHs) derived from proteins decomposition, or mutagenic agents in cooked foods, constitute risks to consumers health.
Biosensors allow the manipulation and real-time monitoring of variables such as pH, temperature, pressure, oxygen, flow, and volatile substances, affecting productivity, profitability and safety of food processing operations. Biosensors can also quantify compounds found in low or high concentrations e.g., sugars, amino acids, alcohols, and acids, as they may become the limiting factors in a production process.
Classification of Biosensors
Biosensors can be classified according to either the nature of the bio receptor element or the principle of operation of the transducer. The main types of transducer used in the development of biosensors can be divided into four groups: 1. Optical, 2. Electrochemical, 3. Mass-Sensitive and 4. Thermometric Each group can be further subdivided into different categories, because of the broad spectrum of methods used to monitor analyte-receptor interactions. The bioreceptor component can be classified into five groups
Enzymes, proteins that catalyze specific chemical reactions
These can be used in a purified form or be present in a microorganism or in a slice of intact tissue. The mechanisms of operation of these bio-receptors can involve: conversion of the analyte into a sensor-detectable product, detection of an analyte that acts as enzyme inhibitor or activator, or evaluation of the modification of enzyme properties upon interaction with the analyte.
Antibodies and antigens
An antigen is a molecule that triggers the immune response of an organism to produce an antibody, a glycoprotein produced by lymphocyte B cells which will specifically recognize the antigen that stimulated its production.
Nucleic acids
The recognition process is based on the complementarity of base pairs i.e adenine and thymine or cytosine and guanine of adjacent strands in the double helix of DNA. These sensors are usually known as genosensors. Alternatively, the interaction of small pollutants with DNA can generate the recognition signal
Cellular structures or whole cells
The whole microorganism or a
specific cellular component, for example, a non-catalytic receptor protein, is used as the bio recognition element.
Biomimetic receptors
Recognition is achieved by the use of receptors, for instance, genetically engineered molecules artificial membranes, or molecularly imprinted polymers (MIP), that mimic a bioreceptor. The most recent investigations in artificial receptors include the application of a combined approach of computer (molecular) modeling and MIP and the application of combinatorial synthesis for the development of new sensing layers.
Components of a Biosensor: The Biosensor System
A biosensor typically consists of a bio-recognition component, biotransducer component, and electronic system which include a signal amplifier, processor, and display. The recognition component, often called a bioreceptor, uses biomolecules from organisms or receptors modeled after biological systems to interact with the analyte of interest. This interaction is measured by the biotranducer which outputs a measurable signal proportional to the presence of the target analyte in the sample. The general aim of the design of a biosensor is to enable quick, convenient testing at the point of concern or care where the sample was procured.
Bioreceptors
In a biosensor, the bioreceptor is designed to interact with the specific analyte of interest to produce an effect measurable by the transducer. High selectivity for the analyte among a matrix of other chemical or biological components is a key requirement of the bioreceptor. While the type of biomolecule used can vary widely, biosensors can be classified according to common types bioreceptor interactions involving: anitbody/antigen, enzymes, nucleic acids/DNA, cellular structures/cells, or biomimetic materials.
Direct detection biosensors are designed in such a way that the biospecific reaction is directly determined in real-time by measuring the physical changes induced by the complex formation. Indirect detection biosensors are those in which a preliminary biochemical reaction takes place and the products of that reaction are then detected by a sensor. Finally, biosensors for bacteria can also be divided into sensors operating in batch and continuous mode.
Direct (label-free) detection of bacteria
Several techniques have been described that allow direct, label-free monitoring of cells at solid-liquid interfaces. These techniques are based on direct measurement of physical phenomena occurring during the biochemical reactions on a transducer surface. Signal parameters such as changes in pH, oxygen consumption, ion concentrations, potential difference, current, resistance, or optical properties can be measured by electrochemical or optical transducers.
Optical biosensors
Optical transducers are particularly attractive for application to direct (label-free) detection of bacteria. These sensors can detect minute changes in the refractive index or thickness which occur when cells bind to receptors immobilized on the transducer surface.
Enzyme-based biosensors
Enzymes are the most commonly used biological components of fiber-optic biosensors. The main reasons are: The large number of reactions they catalyze, the possibility of detecting a broad range of analytes and the different transduction principles that can be used to detect the analyte of interest.
Because enzymes are natural proteins which transform a specific substrate molecule into a product without being consumed in the reaction, they can easily be used for continuous biosensing of a specific compound. Among other advantages enzymes are highly selective and sensitive compared with chemical reactions, they are fairly fast-acting in comparison with other biological receptors, and they can be used in combination with different transduction mechanisms.
Whole-cell-based biosensors
The use of whole living cells, rather than isolated biological components, for biosensor development has several advantages-
- Whole cells are more tolerant of changes in pH or temperature than purified enzymes.
- Some microorganisms (i.e. bacteria, fungi, yeast, etc.) can easily be isolated from natural sources (river water, sediments, soil, activated sludge, etc.).
- A single cell can contain all the enzymes and co-factors needed for detection of the analyte; otherwise, these would have to be provided separately.
- Measurement is frequently possible without extensive preparation of the sample.
- Biosensors can easily be regenerated by letting the cells regrow.
Immunosensors
Immunosensors are an important class of biosensor based on selective bioaffinity interactions between an antibody and a specific compound, or antigen, or a closely related group of antigens. The unique capacity of antibodies to bind specifically the analyte of interest is the key factor in their usefulness in immunosensor design. The higher the affinity of the antibody for the analyte, the better the sensitivity achieved; the selectivity is, on the other hand, a specific property of the antibody applied, rather than depending on the assay format.
Immunosensors are based on the principles of solid phase immunoassays and the assay formats commonly employed in immunosensors. In a direct assay, the antigen is incubated with excess amounts of an immobilized antibody and the interaction is detected. The measured signal is directly proportional to the amount of antigen present.
Nucleic acid biosensors
During recent years the development of genosensor technology has attracted considerable interest, because of its importance in DNA sequencing and the early diagnosis of infection and genetic diseases. The decoding of the human genome has also significantly promoted this development. The favorite transduction principle employed in this type of sensor has been the optical detection of fluorescently labeled oligonucleotides by the use of a competitive assay.
Bio mimetic sensors
The design and synthesis of artificial receptor systems with antibody-like recognition properties have recently attracted much interest. One technique being increasingly adopted for the generation of artificial macromolecular receptors is the molecular imprinting of synthetic polymers (MIP). The molecular imprinting technique consists, basically, in mixing the analyte species, the “templates”, with monomers and a large amount of crosslinker. The template molecules or ionic species form covalent or non-covalent bonded complexes with the functional monomers and the complexes are then polymerized with an excess cross-linking agent, in the presence of a porogenic solvent, to form a resin. On removal of the template species cavities are formed in the polymer matrix; the size, shape, and binding site distribution of these enables selective rebinding of the template from a mixture of chemical species.
Biotransducer
Biosensors can be classified by their biotransducer type. The most common types of biotransducers used in biosensors are 1) electrochemical biosensors, 2) optical biosensors, 3) Electronic biosensors, 4) piezoelectric biosensors, 5) gravimetric biosensors, 6) Pyroelectric biosensors.
Electrochemical
Electrochemical biosensors are normally based on enzymatic catalysis of a reaction that produces or consumes electrons. The sensor substrate usually contains three electrodes; a reference electrode, a working electrode, and a counter electrode. The target analyte is involved in the reaction that takes place on the active electrode surface, and the reaction may cause either electron transfer across the double layer or can contribute to the double layer potential. We can either measure the current at a fixed potential or the potential can be measured at zero current. Note that the potential of the working or active electrode is space charge sensitive and this is often used. Further, the label-free and direct electrical detection of small peptides and proteins are possible by their intrinsic charges using biofunctionalized ion-sensitive field-effect transistors.
Another example, the potentiometric biosensor, gives a logarithmic response with a high dynamic range. Such biosensors are often made by screen printing the electrode patterns on a plastic substrate, coated with a conducting polymer and then some protein is attached. They have only two electrodes and are extremely sensitive and robust. They enable the detection of analytes at levels previously only achievable by HPLC and LC/MS and without rigorous sample preparation.
All biosensors usually involve minimal sample preparation as the biological sensing component is highly selective for the analyte concerned. The signal is produced by electrochemical and physical changes in the conducting polymer layer due to changes occurring at the surface of the sensor. Such changes can be attributed to ionic strength, pH, hydration and redox reactions, the latter due to the enzyme label turning over a substrate. Field effect transistors, in which the gate region has been modified with an enzyme or antibody, can also detect very low concentrations of various analytes as the binding of the analyte to the gate region of the FET cause a change in the drain-source current.
Ion Channel Switch
The use of ion channels has been shown to offer highly sensitive detection of target biological molecules. By embedding the ion channels in supported or tethered bilayer membranes (t-BLM) attached to a gold electrode, an electrical circuit is created. Capture molecules such as antibodies can be bound to the ion channel so that the binding of the target molecule controls the ion flow through the channel. This results in a measurable change in the electrical conduction which is proportional to the concentration of the target.
An Ion Channel Switch (ICS) biosensor can be created using gramicidin, a dimeric peptide channel, in a tethered bilayer membrane. One peptide of gramicidin, with attached antibody, is mobile and one is fixed. Breaking the dimer stops the ionic current through the membrane. The magnitude of the change in electrical signal is greatly increased by separating the membrane from the metal surface using a hydrophilic spacer. Quantitative detection of an extensive class of target species, including proteins, bacteria, drug, and toxins has been demonstrated using different membrane and capture configurations.
Others Piezoelectric sensors utilise crystals which undergo elastic deformation when an electrical potential is applied to them. An alternating potential (A.C.) produce a standing wave in the crystal at a characteristic frequency. This frequency is highly dependent on the elastic properties of the crystal, such that if a crystal is coated with a biological recognition element the binding of a (large) target analyte to a receptor will produce a change in the resonance frequency, which gives a binding signal. In a mode that uses surface acoustic waves (SAW), the sensitivity is greatly increased.
Biosensors used for the detection of bacteria
Salmonella
Salmonella are Gram-negative bacteria naturally found in the gastrointestinal tract of warm-blooded animals and humans. When out of their natural habitat, these bacteria can survive in water and food products.
The consumption of contaminated food products causes diseases such as enteric fever and salmonellosis.
For the rapid detection of Salmonella, piezoelectric antigen-antibody biosensors are used. These sensors may apply the Langmuir-Blodgett (LB) monolayer method, which is based on the immobilization of amphiphilic antibodies. The antibodies are captured and distributed uniformly in a liquid having an affinity to the antibody. Antibodies can be monoclonal or polyclonal; however, the use of monoclonal antibodies is ideal because it offers greater specificity.
When submerging a supported probe with a polar and a nonpolar surface, little interaction with the antibody occurs. However, binding between the antibody and the polar surface will occur when the support emerges from the liquid. The support will have transduction resonance properties, i.e., when affinity with antigen takes place, the resonance frequency will be affected due to increased mass in the crystal support.
The system reaches equilibrium in the surface to reduce vibrations generated by external factors, in such a way that frequency and amplitude changes are only caused by mass variations in the crystal. This method was studied using different biosensor sizes and it was concluded that better changes in resonance frequency were achieved with small sensors. In these sensors, the resonance frequency decreases with an increase in the number of pathogen microorganisms bound to the antibody.
Listeria monocytogenes
Listeria monocytogenes, a Gram-positive, flagellate microaerophilic coccobacillus, can cause listeriosis associated to the consumption of fresh and processed meats, The disease produces little morbidity but important mortality in humans and animals, with symptoms such as muscle pain, nausea, diarrhea, and chills.
A fiber-optic biosensor can be used for the detection of Listeria monocytogenes. It is based on the measurement of fluorescent light generated by a wave when there is a change in resonance. The biosensor uses monoclonal fluorescent antibodies that generate excitation of atoms and wave production when bound to the pathogen. The biosensor is made of polystyrene support that immobilizes the antibodies.
The antibodies are obtained by inducing the immune response of rabbits after inoculating with Listeria monocytogenes for three months. The quick response from this biosensor and the specificity against L. monocytogenes were verified in the presence of bacterial pollutants common in foods such as E. coli, Enterococcus faecalis, and S. enteric. Its response in cold (4°C) and salty conditions showed lower reaction rates due to “stress” of the monoclonal antibodies at low temperatures.
Toxin Alert (Ontario, Canada) is a visual diagnostic system that incorporates antibodies in a polyethylene-based plastic packaging and is capable of detecting Salmonella sp., Campylobacter sp., E. coli 0517 and Listeria sp.
Biosensors used for the detection of heavy metals
Heavy metals are toxic substances that accumulate in the organism and cause metabolic alterations since there is no way of metabolizing or excreting them. We may accumulate heavy metals when eating foods of animal origin since animals have greater contact with poorly treated water, can graze close to industries, and even eat foods treated with water contaminated with heavy metals. Diseases caused by the ingestion of heavy metals include cardiovascular and respiratory problems, infertility, irritations, inhibition of some hormonal activities, malfunction of the principal organs, and death.
Devices have been designed to determine the concentration of heavy metals such as arsenic, cadmium, mercury, and lead, in water and soil samples. These devices incorporate genetically modified microorganisms and enzymes such as urease, cholinesterase, glucose oxidase, alkaline phosphatase, ascorbate oxidase and peroxidase, incorporated to electrochemical and optical transduction systems.
Biosensor to early determination of meat ultimate pH
A patented method of rapidly determining the ultimate pH from the approximate glycolytic potential of muscles of slaughtered animals has been devised. The method is based on the rapid hydrolysis of muscle glycogen to glucose by the enzyme amyloglucosidase and subsequent measurement of the liberated glucose. In acetate buffer at pH 4.5, glucose concentration can be determined in 30 s with domestic meters for diabetes control.
The meter response differed from that of glucose in the blood but was linear with concentration. In the 24 h following slaughter, a decrease in glycogen, as determined by glucose, occurred in parallel with the decline in pH. At the same time, lactate progressively accumulated as expected. Values for the approximate glycolytic potential and (by calibration) ultimate pH, were obtained on prerigor muscle within 7 min of muscle sampling in an industrial environment.
Many qualities of meat depend on its pH. Generally, meat in the pH range of 5.4 to 5.6 has the most desirable for table cuts. Higher values, which can reach pH 6.9, resulting in several defects, the most obvious being its colour, which becomes progressively darker as pH increases. As a result, high pH meat is sometimes called ‘dark-cutting’ or ‘DFD’, dark, firm, and dry, referring to the meat’s physical properties. The microbiological stability of high pH meat is poor, tenderness is more variable, and the cooked flavour is inferior (Braggins, 1996; Gill & Newton, 1981; Simmons, Auld, Thomson, Cairney, & Daly, 2000).
For a muscle to achieve a pH around 5.5, it must contain a sufficient concentration of glycogen at slaughter. When the muscle becomes anaerobic after slaughter, glycogen is converted to lactate by way of the glycolytic pathway. Hydrogen ions are produced at the same time, causing the pH to fall. If the glycogen concentration is limited—by factors discussed below-pH decline is arrested at higher values than 5.5, resulting in dark-cutting meat. Sometimes there is more than enough glycogen to achieve a pH around 5.5. In such cases, the pH does not fall much lower than 5.4, possibly because the glycolytic enzymes are inactivated by the acidic conditions. In this situation, residual glycogen remains a condition not found when the ultimate pH is above about 5.7.
Detection of chemical and veterinary drug residues in animal foods
Rapid methods and automation for the detection and characterisation of chemical and veterinary drug residues in foods of animal origin constitutes a dynamic area in food processing and is experiencing important developments mainly from the standpoint of food safety. Residues from these substances may be present in edible tissues, milk, and eggs for human consumption and may exert different levels of toxicity on consumers when consuming them. Thus, easy, rapid and sensitive tests are needed for effective at-line use. This manuscript is presenting the latest developments for the rapid detection of chemical and veterinary drugs residues in foods of animal origin.
Biosensors as indicators of product acceptability
Food quality involves nutritional and organoleptic characteristics important for consumers such as freshness, appearance, taste, and texture. The food sensory basis is essential for the industry. A method to determine food freshness is by assessing the food composition of products such as meats, fish, fruits, and vegetables. During storage, compounds that provide aroma and abnormal flavors or may be harmful to the consumer may be synthesized, indicating in most cases microbial growth and insufficient food safety.
Biosensors that use whole cells or enzymes have been used for the detection of alcohol. Alcohol enzymatic biosensors described in the literature are mainly based on alcohol dehydrogenase and alcohol oxidase, and less commonly on catalase. Ethanol biosensors using alcohol oxidase as biorecognition element are the most abundant. Alcohol oxidase is an oligomeric enzyme responsible for the oxidation of low molecular weight primary alcohols, using molecular oxygen as the electron acceptor and producing acetaldehyde and hydrogen peroxide. Due to the strong oxidizing character of oxygen, the oxidation of alcohols by alcohol oxidase is irreversible. The reaction may be followed by measuring either O2 decline or H2O2 increase using optical or electrochemical detections.
Utilisation of active and intelligent packaging systems for meat and muscle-based products
Interest in the use of active and intelligent packaging systems for meat and meat products has increased in recent years. Active packaging refers to the incorporation of additives into packaging systems to maintain or extend meat product quality and shelf-life. Active packaging systems discussed include oxygen scavengers, carbon dioxide scavengers and emitters, moisture control agents and antimicrobial packaging technologies. Intelligent packaging systems are those that monitor the condition of packaged foods to give information regarding the quality of the packaged food during transport and storage.
The potential of sensor technologies, indicators (including integrity, freshness, and time-temperature (TTI) indicators) and radio frequency identification (RFID) are evaluated for potential use in meat and meat products. Recognition of the benefits of active and intelligent packaging technologies by the food industry, development of economically viable packaging systems and increased consumer acceptance is necessary for the commercial realisation of these packaging technologies.
Intelligent packaging
Definition of intelligent packaging (and one to which these authors shall adhere for this review) is provided by the European study ‘Evaluating Safety, Effectiveness, Economic- environmental Impact and Consumer Acceptance of Active and Intelligent Packaging (ACTIPAK-FAIR CT 98-4170, 1999–2001) as ‘systems that monitor the condition of packaged foods to give information about the quality of the packaged food during transport and storage’.
Sensors
The use of these systems is generally envisaged in terms of incorporation into established packaging techniques such as MAP and vacuum packaging. MAP is an extremely important packaging technique used extensively for the distribution, storage, and display of meat products in markets with a controlled cold distribution chain. The most important (non-inert) gases in MAP products are oxygen and carbon dioxide and their headspace partial pressures serve as useful indicators of the quality status of a meat product. The profiles of oxygen and carbon dioxide can change over time and are influenced by product type, respiration, packaging material, pack size, volume ratios, storage conditions, package integrity, etc.
Several analytical techniques are available to monitor gas phases in MAP products. Instrumental techniques such as GC and GC/MS require breakage of package integrity and are time-consuming and expensive. Portable headspace oxygen and/or carbon dioxide gas analysers use ‘minimally destructive’ techniques (packages can be resealed) but tend not to apply to real-time, on-line control of packaging processes or large scale usage. An optical sensor approach offers a realistic alternative to such conventional methods.
A sensor is defined as a device used to detect, locate or quantify energy or matter, giving a signal for the detection or measurement of a physical or chemical property to which the device responds (Kress-Rogers, 1998).
Gas sensors
Gas sensors are devices that respond reversibly and quantitatively to the presence of a gaseous analyte by changing the physical parameters of the sensor and are monitored by an external device. Systems presently available for gas detection include amperometric oxygen sensors, potentiometric carbon dioxide sensors, metal oxide semiconductor field-effect transistors, organic conducting polymers and piezoelectric crystal sensors.
Sensors are usually comprised of a solid-state material, which operates on the principle of luminescence quenching or absorbance changes caused by direct contact with the analyte. These systems provide a non-invasive technique for gas analysis through translucent materials and as such are potentially suitable for intelligent packaging applications. The solid-state sensor is inert and does not consume analyte or undergo other chemical reactions
Fluoresence-based oxygen sensors
The active component of a fluorescence-based oxygen sensor normally consists of a long-delay fluorescent or phosphorescent dye encapsulated in a solid polymer matrix. The dye-polymer coating is applied as a thin film coating on a suitable solid support.
Molecular oxygen, present in the packaging headspace, penetrates the sensitive coating through simple diffusion and quenches luminescence by a dynamic, i.e. collisional mechanism. Oxygen is quantified by measuring changes in luminescence parameters from the oxygen-sensing element in contact with the gas or liquid sample, using a pre-determined calibration. The process is reversible and clean: neither the dye nor oxygen is consumed in the photochemical reactions involved, no by-products are generated and the whole cycle can be repeated.
Materials for oxygen sensors must meet strict sensitivity and working performance requirements if they are to prove suitable for commercial intelligent packaging applications. Materials using fluorescent complexes of ruthenium, phosphorescent palladium (II) and platinum(II) porphyrin complexes and related structures have shown promise as oxygen sensors.
OxySense (http://www.oxysense.com) is the first commercially available fluorescence quenching sensor system for measurement of headspace or dissolved oxygen in transparent or semi-transparent, sealed packages. The system uses an oxygen sensor (O2xyDot) placed in the package before filling and is non-destructive, rapid (measurements take less than 5 s) and able to withstand pasteurisation temperatures without loss of sensitivity.
Indicators
Defined as a substance that indicates, the presence or absence of another substance or the degree of reaction between two or more substances using a characteristic change, especially in colour. In contrast with sensors, indicators do not comprise receptor and transducer components and communicate information through direct visual change.
Integrity indicators
Alternative approach to package-destructive, quality assurance techniques is the use of non-invasive indicator systems as part of an MA package. Such systems usually provide qualitative or semi-quantitative information through visual colorimetric changes or comparison with standard references. The majority of indicators have been developed for package integrity testing, an essential requirement for the maintenance of quality and safety standards in the packaging of meat products.
The most common cause of integrity damage in flexible plastic packages is associated with leaking seals. Permanent attachment of a leak indicator or sensor (i.e. visual or optochemical) to a package appears to hold the most promise in the development of integrity detection during package integrity throughout the production and distribution chain.
Development of integrity detection for packaged foods has focused on visual oxygen indicators in MAP foods (as opposed to those oxygen sensors, which are also applicable to integrity testing). Except for high oxygen content MA packaging of fresh meat (primarily to enhance colour) many foods are packaged in low (0–2%) oxygen atmospheres. In such cases, leaks normally result in a significant increase in oxygen concentration. Many visual oxygen indicators consisting mainly of redox dyes have been patented.
Some companies have produced oxygen indicators, the main application of which has been for the confirmation of proper functioning of oxygen absorbers (an active packaging function). Trade names of such devices have included Ageless Eye, Vitalon, and Samso- Checker.
Freshness indicators
Freshness indicators provide direct product quality information resulting from microbial growth or chemical changes within a food product. Microbiological quality may be determined through reactions between indicators included within the package and microbial growth metabolites. The formation of different potential indicator metabolites in meat products is dependent on the product type, associated spoilage flora, storage conditions, and packaging system. Several marker metabolites associated with muscle food products exist upon which indicator development may be based.
Changes in the concentration of organic acids such as nbutyrate, L-lactic acid, D-lactate, and acetic acid during storage offer potential as indicator metabolites for many meat products. Ethanol, like lactic acid and acetic acid, is an important indicator of fermentative metabolism of lactic acid bacteria. Biogenic amines such as histamine, putrescine, tyramine, and cadaverine have been implicated as indicators of meat product decomposition.
Hydrogen sulphide, a breakdown product of cysteine, with intense off-flavours and low threshold levels is produced during the spoilage of meat and poultry by a number of bacterial species. It forms a green pigment, sulphmyocin, when bound to myoglobin and this pigment formed the basis for the development of an agarose-immobilised, myoglobin- based freshness indicator for unmarinated broiler pieces. The indicator was not affected by the presence of nitrogen or carbon dioxide and offers potential.
Time-temperature indicators
A time-temperature indicator or integrator (TTI) may be defined as a device used to show a measurable, time-temperature dependent change that reflects the full or partial temperature history of a food product to which it is attached. Operation of TTIs is based on mechanical, chemical, electrochemical, enzymatic or microbiological change, usually expressed as a visible response in the form of a mechanical deformation, colour development or colour movement.
Diffusion-based TTIs. The 3M Monitor Mark_ (3M Company, St. Paul, MN, USA) is an indicator depends on the diffusion of a coloured fatty acid ester along with a porous wick made of high-quality blotting paper. The measurable response is the distance of the advancing diffusion front from the origin. The useful range of temperatures and the response life of the TTI are determined by the type and concentration of ester. Another diffusion-based TTI, Freshness Check, produced by the same company incorporates a viscoelastic material that migrates into a diffusively light-reflective porous matrix at a temperature-dependent rate. This causes a progressive change in the light transmissivity of the porous matrix and provides a visual response.
Enzymatic TTIs. The VITSAB TTI (VITSAB A.B., Malmo, Sweden) is based on a colour change induced by a drop in pH resulting from the controlled enzymatic hydrolysis of a lipid substrate. The indicator consists of two separate compartments containing an aqueous solution of lipolytic enzymes and another containing the lipid substrate suspended in an aqueous medium and a pH indicator mix. Different enzyme-substrate combinations are available to give a variety of response lives and temperature dependencies.
Activation of the TTI is brought about by mechanical breakage of a seal separating the two compartments and may be done manually or by on-line automation. Hydrolysis of the substrate causes a drop in pH and a subsequent colour change in the pH indicator from dark green to bright yellow. Visual evaluation of the colour change is made by reference to a five-point colour scale. Check Point labels are the latest TTIs developed by VITSAB, which comprise a label type designed to create a better subjective reading response for users and offer direct application to poultry and ground beef products. VITSAB, in conjunction with British Airways, has also recently developed a TTI system (Flight 17 Smart Label) that allows airline personnel to check the status of perishable pre-prepared foods.
Polymer-based TTIs. Lifelines Freshness Monitor and Fresh-Check TTIs (Lifelines Technology Inc., Morris Plains, NJ, USA) are based on temperature-dependent polymeri-sation reactions in which diacetylene crystals polymerise via 1,4 addition polymerisation to a highly coloured polymer. Resulting changes in reflectance can be measured by scanning with a laser optic wand. The Fresh-Check consumer version uses a circular label in which the colour of the inner circle is compared to that of an outer circle to establish use-by status.
You may also be interested in our recent useful publication:
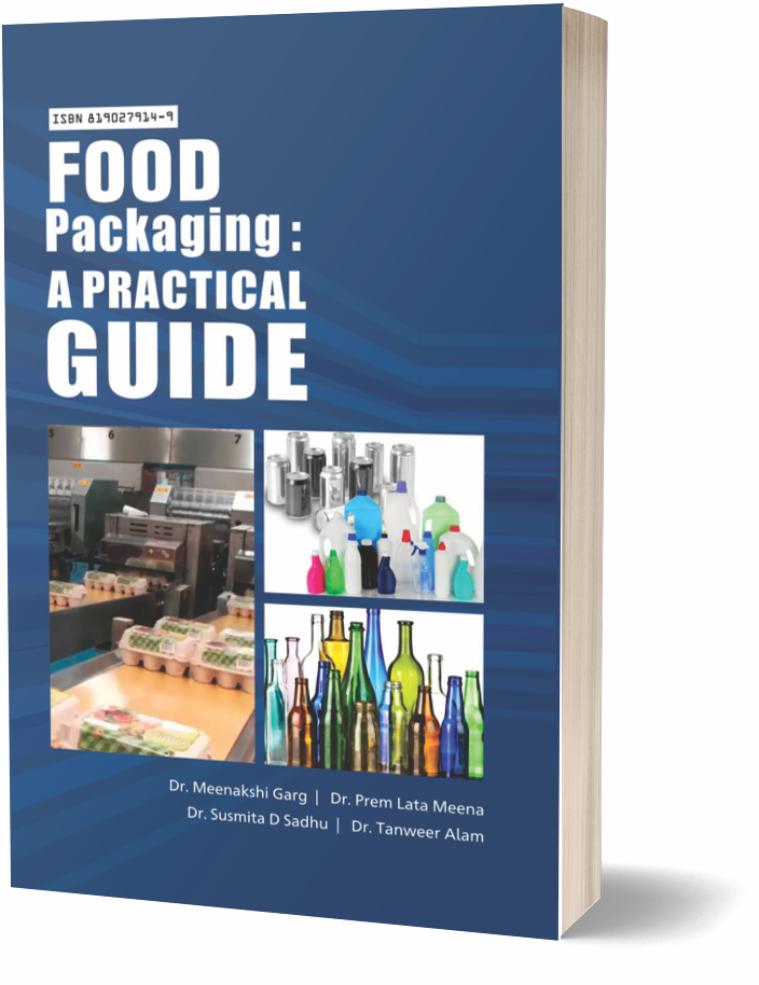
PP 248 | Price Rs.250
Food Packaging: A Practical Guide
Edited & Compiled By:
Dr Meenakshi Garg, Dr Prem Lata Meena
Dr Susmita D Sadhu and Prof. Tanweer Alam
Packaging is described as a “Complex, dynamic, scientific, artistic and controversial segment of business”. Product packaging often serves as the first impression of a brand for a consumer. There are various types of tests performed for all kinds of packaging materials.
The book covers all the important test methods for qualitatively and quantitatively analysing the packaging materials including plastic film, paper, paperboard, tin and glass. The detailed explanation of each and every described test methods in the book would definitely be beneficial for graduate and post graduate students, teaching and R & D professionals of Packaging Industry.
Send your orders along with the Rs.250 + 50 (Postal Charges)
The Computype Media,
208 IJS Place, Delhi Gate Bazar, Darya Ganj, New Delhi-110002 (India)
Phone: 011-23284148 w Email: computype.media@gmail.com
References
- Fidel Toldra and Milagro Reig, 2010. Methods for rapid detection of chemical and veterinary drug residues in animal foods.
- Jeyson G., Perenguez-Verdugo Facultad de Ingeniería, 2001. Biosensors Applications in Agri-food Industry Liliana Serna-CockKress-Rogers, Erika. Instrumentation and Sensors for the Food Industry. Ed. Christopher Brimelow. Oxford: Butterworth- Heinemann.
- J. P. Kerry, M. N. O’Grady, S.A. Hogan Past, 2006. Current and potential utilisation of active and intelligent packaging systems for meat and muscle-based products: A review. Meat Science, 74:113-130.
Kumar, J. & D’Souza, S., 2010. An optical microbial biosensor for detection of methyl parathion using - Sphingomonas sp. immobilized on microplate as a reusable biocomponent. Biosensors and Bioelectronics, 89:213-214.
- Pathirana, S., Barbaree, J., Chin, B., Hartell, M., Neely, W. & Vodyanoy, V., 2000. Rapid and sensitive biosensor for Salmonella. Biosensors and Bioelectronics, 78:149-151.
- Trivedi, U., Lakshminarayana, D., Kothari, I., Patel, N., Kapse, H., Makhija, K., Patel, P. & Panchal, C., 2009. Potentiometric biosensor for urea determination in milk. Sensors and Actuators B: Chemical, Vol. 140, No. 1, pp. 260–266.