Edible films and coatings help in encompassing the shelf lives of various products. Increasing consciousness about ecological problems are among the major factors behind food producers’ increasing focus on recyclable packaging solutions. Some successful outcomes of similar efforts on fruits, vegetables, and other food products are reviewed here by Preeti Shukla, Suresh Bhise and S. S. Thind.
Table of Contents
The application of edible films and coatings can readily improve the physical strength of food products, reduce particle clustering and improve visual and tactile features on the product surface (Cuq et al., 1995). It can protect food products from moisture migration, microbial growth on the surface, light-induced chemical changes, oxidation of nutrients, etc (Kester and Fennema, 1986). You can explore more about the role of edible films in food quality through the article on edible films and coatings enhancing food quality and shelf life.
Generally, edible films and coatings function as a barrier against gases or vapor and as carriers of active ingredients, such as antioxidants, flavours, fortified nutrients, colorants, antimicrobial agents, or spices (Gennadios and Weller, 1990; Guilbert et al., 1995). The purpose of edible films is to provide mechanical integrity or handling characteristics of the food, and a selective barrier to various gases, moisture, aroma, and lipids. Besides their barrier properties, edible films and coatings may control adhesion, cohesion, and durability, and improve the appearance of coated foods.
The benefits of edible films and coatings for food products
There are several advantages associated with an edible coating like (1) they may be eaten by the consumer along with food (2) their use could reduce the waste and solve the solid disposal problem (3) they could enhance the organoleptic, mechanical or nutritional properties of food, (4) they can reduce the cost by utilizing by-products e.g., whey (Guilbert, 1995).
Baldwin et al. (1991) reported that the use of an edible coating to preserve the quality of minimally processed fruits, vegetables and nuts are desirable. The edible coating generally stabilizes the products thereby increasing the shelf life. Specifically, the coatings have the potential to reduce moisture loss, restrict oxygen entrance, lower respiration, retard ethylene production, seal in flavour volatiles and carry additives that retard discoloration and microbial growth in various food products.
To learn more about innovative food preservation methods, check out how packaging extends the shelf life of food products.
How edible coatings can improve the shelf life of fruits and vegetables
The coating of food products with some agents may also protect against surface spoilage of food products. The edible coating is usually used for coating and improving the shelf life of fruits as studied by several authors. These protecting functions are aimed to enhance the quality of food products, resulting in shelf life extension and safety improvement (Gennadios and Weller, 1990). Although no work has been done on the use of edible films and coating in improving the shelf life of jaggery and its products, some successful outcome of similar efforts on fruits, vegetables, and other food products is reviewed here.
The effect of wrappers and wax coatings on the apple storage at room temperature has been studied by Singh (1973). Polythene was found to be the best wrapping material, as indicated by least fruit rotting, weight loss and shriveling. Alleynev et al. (2000) studied an alternative formulation for coating apples with Candelilla-shellac. A gloss of all coated fruits decreased with time, although shellac coated fruit lost less gloss over the 21-days storage period. It was observed that the effect of active edible polysaccharide coating and interactions between solution coating compounds. The addition of Ca2+ to the nisin-sa mixture was effective in increasing nisin release from the films. The wax-coated paper was better packaging material than polyethylene for the storage of paneer.
For example, innovative edible biofilms are being used to combat food waste and improve product freshness.
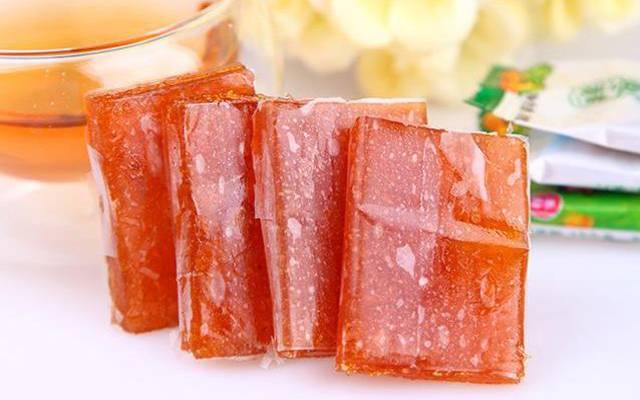
Composite film coatings vs. pure protein or polysaccharide films
Composite film coating is more effective than pure protein or polysaccharide films as reported by Kester and Fenema, (1986). Nisin is one of the most heavily investigated bacteriocins in antimicrobial edible film studies. It can be incorporated into the film solution or applied directly to the film surface after casting. Various nisin containing protein-based films (e.g. whey protein, sodium caseinate, corn zein, wheat protein, and soy protein) have been assessed for antimicrobial activity against pathogens. Whey protein isolate/plasticizer formulation of the edible coating was found to be effective as a source of glaze in the confectionery industry (Lee et al., 2002). Whey protein films and coatings are excellent aroma and oxygen barrier, moisture barrier as well as gloss enhancers (McHugh and Krochta, 1994; Lee et al., 2002).
This film can be applied over or between foods by immersion, spraying, or panning. When food-grade proteins and other food-grade additives (e.g. plasticizers, surfactants, acid or base, salts, and enzymes) are used and only protein changes due to heating, pH modification, salt addition, enzymatic modification, and water removal, the resulting film or coating is edible. This type of material unlike synthetic polymers comes from natural sources and is biodegradable i.e. they can be degraded completely by microorganisms in a composting process to only natural compounds such as carbon dioxide, water, methane, and biomass.
Whey protein isolate/plasticizer formulation of the edible coating was found to be effective as a source of glaze in the confectionery industry (Lee et al., 2002). When food-grade proteins and other food-grade additives (e.g. plasticizers, surfactants, acid or base, salts, and enzymes) are used, only protein changes due to heating, pH modification, salt addition, enzymatic modification, and water removal, the resulting film or coating is edible. This type of material unlike synthetic polymers comes from natural sources and is biodegradable i.e. they can be degraded completely by microorganisms in a composting process to only natural compounds such as carbon dioxide, water, methane, and biomass.
To explore trends in the dairy sector, read the role of whey proteins in food packaging and quality.
Edible film and coating formation
There are many methods to produce whey protein-based edible films, such as heating and irradiation. A process of protein cross-linking is necessary to obtain a flexible, easy to handle film. The resulting film properties are affected by the amino acid composition, distribution and polarity, conditions affecting the formation of ionic cross-linking between amino and carboxyl groups, presence of hydrogen bonding, intramolecular and intermolecular disulfide bonds. However, the formation of whey protein-based films mainly involved heat denaturation in aqueous solution at 75-100°C, which produces intermolecular disulfide bonds, which might be partly responsible for film structure (www.enwikipedia.org).
If you’re interested in understanding more about biodegradable packaging, read new sustainable packaging material technologies.
Heat treatment promotes water insolubility, which may be beneficial to maintain film and food integrity. There are reports where WPI films plasticized with sorbitol (S) or glycerol (G) was slightly sweet and adhesive. On the other hand, the textural impact of glycerol plasticized whey protein isolate (WPI) films could be reduced if film thickness was reduced to about 23 ìm, as tested on crackers and melted cheese. Texture perception of WPI films may also be reduced by making them more soluble so that they dissolve readily in the mouth during mastication (www.enwikipedia.org).
Plasticizers
Plasticizers are the substances that decrease activation energy for the diffusion of gases and vapors through the film and can decrease elasticity and cohesion. Commonly used plasticizers in film systems are monosaccharides (glucose), disaccharides (sucrose), oligosaccharides, polyols (sorbitol, glycerol, mannitol, glycerol derivatives, and polyethylene glycols), and certain lipids and derivatives (phospholipids, fatty acids, surfactants, etc.). Lipids or waxes may interfere with polymer chain to chain interaction and/or provide flexible domains within the film leading to a reduction of film strength and increase of film flexibility in whey proteins. Water also acts as plasticizer for edible films and coatings, and hydrophilic plasticizers generally attract additional water. Plasticizers such as glycerol and propylene glycol have shown a secondary role since they enhanced significantly the formation of cross-links within milk proteins (e.g. caseinates).
Whey protein films require plasticizers at 10 percent to 60 percent on dry basis, depending on the rigidity of the polymer. The influence of an additive, including plasticizers or functional carriers, on edible film properties, will depend on its concentration, chemical structure, degree of dispersion and the extent of its interaction with the polymer. One way to decrease the amount of plasticizer is to reduce the polymer molecular weight to reduce intermolecular forces along the polymer chains, increasing polymer chain end groups and polymer-free volume.
It has been shown that hydrolyzed WPI has greater solubility and emulsifying activity, while allergenicity of WPI is reduced due to the hydrolysis process. The solubility of (hydrolyzed WPI edible films (30-75 percent soluble protein) at 5 and 10 percent degree of hydrolysis was found higher than that shown by unhydrolyzed WPI (about 3 percent) under equal plasticizer (G) concentration. Insoluble films may be used on high-moisture foods, while readily soluble films can find use in water-soluble pouches.
Additives can enhance food quality and safety
Edible films and coatings can carry various active agents, such as emulsifiers, antioxidants, antimicrobials, nutraceuticals, flavors, and colorants, thus enhancing food quality and safety, up to the level where the additives interfere with physical and mechanical properties of the films. Emulsifiers are surface-active agents of amphiphilic nature able to reduce the surface tension of the water-lipid interface or the water-air surface. Emulsifiers are essential for the formation of protein or polysaccharide films containing lipid emulsion particles. They also modify surface energy to control the adhesion and wet ability of the film surface. Antioxidants and antimicrobial agents can be incorporated into film-forming solutions to achieve active packaging or coating functions.
To learn about advancements in safe food packaging, explore the future prospects of sustainable packaging.
They provide additional active functions to the edible film and coating system to protect food products from oxidation and microbial spoilage, resulting in quality improvement and safety enhancement. When nutraceutical and pharmaceutical substances are incorporated into edible films and coatings, the system can be used for drug delivery purposes. Incorporated flavors and colorants can improve the taste and the visual perception of quality, respectively. Because of the various chemical characteristics of these active additives, film composition should be modified to keep a homogeneous film structure when heterogeneous additives are incorporated into the film-forming materials.
Materials Used for Edible Films and Coatings
The selection of materials for manufacturing edible film is based on its properties, to act as a barrier to moisture and gases and mechanical strength. Types of edible coating are categorized based on base material used for the preparation of edible coating. Various materials used for the manufacture of edible coating are shown in Table1.
Protein-based films and coatings
The inherent properties of proteins make them excellent starting materials for films and coatings. The distribution of charged, polar and non-polar amino acids along the protein chain creates chemical potential Proteins have multiple sites for chemical interaction as a function of their diverse amino acid functional groups, which can allow for property improvement and tailoring. Chemical changes can improve the stability of films and coatings. Cross-linked protein films are often more stable than their polysaccharide-based counterparts and have longer lifetime Protein-based films and coatings are also biodegradable and compostable. As they degrade, they provide a source of nitrogen, which contributes to a fertilizer benefit not available with other non-protein-based films and coatings. Finally, there is emerging evidence that the bioactive peptides produced upon the digestion of proteins (dairy sources in particular) have antihypertensive and radical scavenging health benefits.
Composition of films and coatings
Films and coatings may be made from proteins of both animal and plant origin. Protein-based films and coatings are prepared from solutions comprised of three main components: protein, plasticizer, and solvent. The properties of the final film are affected by the intrinsic properties of the film or coating components and extrinsic processing factors Intrinsic properties of proteins include amino acid composition, crystallinity (of the protein and/or plasticizer), hydrophobicity/ hydrophilicity, surface charge, pI, molecular size, and three-dimensional shape. The presence of cysteine allows for potential disulfide bridge formation, as noted for beta-lactoglobulin. High concentrations of leucine, alanine and other nonpolar amino acids can create hydrophobic proteins. Extrinsic factors include processing temperature, drying conditions, pH, ionic strength, salt-type, relative humidity during processing and storage, shear and pressure.
Whey Protein
Whey is a byproduct of the cheese-making process, and whey proteins are technically defined as those that remain in the milk serum after coagulation of the caseins at a pH of 4.6 and a temperature of 20°C. Whey protein is comprised of several individual proteins, with beta-lactoglobulin, alpha-lactalbumin, bovine serum albumin, and immunoglobins being the main proteins Whey proteins differ from caseins in that their net negative charge is evenly distributed over the protein chain. The hydrophobic, polar and charged amino acids are also uniformly distributed. Consequently, the proteins fold so that most of the hydrophobic groups are buried within the whey protein molecule. Extensive self-association does not occur under neutral conditions, as seen among casein proteins The protein interactions that occur between chains determine film network formation and properties.
Collagen
Collagen is a fibrous, stromal protein extracted from connective tissue, tendons, skin, bones and vascular system, which are waste products of meat processing. Collagen is made from three parallel alpha-chains, which combine to make a triple-stranded superhelical structure. The amino acid sequence of collagen is dominated by a repeating trio of Gly-X-Y residues.
Gelatin
Gelatin is formed when collagen is exposed to a mild heat treatment under acidic or alkaline conditions. In this process, collagen is partially denatured, but upon cooling, partially reforms the triple helix structure, though unstructured domains also exist.
The resulting protein material is referred to as gelatin. Gelatin contains a large amount of proline, hydroxyproline, lysine, and hydroxylysine, which can react in an aldol-condensation reaction to form intra- and intermolecular cross-links among the protein chains. Gelatin is used in the food industry to thicken and texturize foods, because of its good gelling properties. It also has excellent foaming properties that translate to it being a good edible film former. Gelatin has been used as a coating by the food and pharmaceutical industry for years.
Egg Albumen
Egg albumen is the second major component of liquid egg white, water being the first, and makes up approximately 10% of the total liquid egg white weight. Within egg albumen, there are five main protein fractions: ovalbumin, ovotransferrin, ovomucoid, ovomucin, and lysozyme. Differential scanning calorimetry analysis shows three main endotherms that occur during heating of egg white protein that corresponds to the denaturation of ovotransferrin, lysozyme, and ovalbumin. Egg albumen proteins are thermolabile and form strong heat-set gels.
During heat denaturation, egg white proteins form stable intermolecular beta-sheet structures. Between ovalbumin, ovotransferrin, and lysozyme. Above 60°C, egg white proteins unfold, exposing their internal sulfhydryl groups that can affect disulfide bond formation, and surface hydrophobicity increases.
Soy Protein
Soy protein is comprised of a mixture of globular proteins. Approximately 90% of soy proteins separate into either a 2S, 7S, 11S or 15S fraction, based on molecular weight and sedimentation coefficient. The two main globular proteins are beta-conglycinin (7S globulin) and glycinin (11S globulin), which make up 37% and 31% of the soy proteins, respectively. Conglycinin (140–170 kDa) consists of various combinations of three subunits, which are heavily glycosylated.
As with other film-forming proteins, glycinin is known to be a gelling agent, emulsifier and foaming agent. Heat and alkaline conditions can denature soy proteins, affecting film formation. Similar to beta-lactoglobulin (whey protein), glycinin (11S protein) forms intermolecular disulfide bonds when denatured, which bonds affect the tensile properties of a formed film. Soy protein association and stability is dependent on the pH and ionic strength.
Corn Zein
Zein protein from corn has some unique characteristics compared to most other agricultural proteins used for edible films and coatings. Zein possesses a high percentage of nonpolar amino acids and low proportions of basic and acidic amino acids. The three primary amino acids in corn zein protein are glutamine (21–26%), leucine (20%) and proline (10%). Consequently, corn zein protein is insoluble in water, a characteristic that affects the barrier properties of its films.
The two major fractions of zein are alpha-zein and beta-zein. Alpha-zein is soluble in 95% ethanol, and makes up approximately 80% of the total prolamines present in corn, while beta-zein is soluble in 60% ethanol. A helical secondary structure dominates zein proteins. When formed into films, zein is glossy, tough and greaseproof, with a low water vapor permeability compared to most other agriculturally-based protein films. Zein has been commercially used as a coating for medical tablets and has the potential to be used in biodegradable packaging.
Function and Applications of Protein-Based Films
Based on the required tensile, barrier and appearance characteristics and properties, protein-based edible films for specific applications can be designed. At the current state of edible film technology, the best application potential lies in protective coatings for foods. When applied to the surfaces of foods as a coating, protein-based edible films can protect food from chemical or microbial damage, thus lengthening product shelf life and maintaining high product quality.
Most protein-based films provide excellent barriers to oxygen. This characteristic has been utilized to effectively protect high-fat foods, which are known to form rancid off-flavors, due to oxidation. As barriers to mass transfer of carbon dioxide, protein-based coatings have been found to increase the shelf life of eggs and fresh-cut fruits. Egg quality drops over time as carbon dioxide migrates out of the shell, causing changes to the internal pH. These pH changes can deleteriously affect color and yolk quality. The shelf life of whey protein-coated Grade A eggs could be extended one week longer than uncoated eggs when stored under ambient laboratory conditions. In the case of fresh-cut produce, browning and ripening times are major factors determining product shelf life.
Protein-based edible coatings that have moderate oxygen, carbon dioxide, and water vapor permeability can be applied to the surfaces of fresh-cut produce to extend product shelf life by delaying ripening, inhibiting enzymatic browning, reducing water loss, and minimizing aroma loss. whey protein coatings significantly delayed browning in fresh-cut Macintosh apples and Russet potatoes. Edible films used to protect fresh-cut produce have advantages over modified atmosphere packaging or treating the cut surfaces with ascorbic acid (which inhibits browning).
Polysaccharide and Gum-Based Edible Films and Coatings
Polysaccharide gums are hydrocolloids of considerable molecular weight and are water-soluble. They dissolve in and form intensive hydrogen bonds with water. Because of the size and configuration of their molecules, these polysaccharides can thicken and/or gel aqueous solutions as a result of both hydrogen bonding between polymer chains and intermolecular friction when subjected to shear are hydrocolloids of considerable molecular weight, and are water-soluble. They dissolve in and form intensive hydrogen bonds with water.
Because of the size and configuration of their molecules, these polysaccharides can thicken and/or gel aqueous solutions as a result of both hydrogen bonding between polymer chains and intermolecular friction when subjected to shear. Gums dissolve in water through the formation of solvent–polymer hydrogen bonds; in solution, polymer molecules may arrange themselves into an ordered structure, called a micelle that is stabilized or fortified by intermolecular hydrogen bonds The micelle traps and immobilizes water and, depending on the extent of the intermolecular association, the water is either thickened, as measured by a parameter called viscosity, or converted into a gel that possesses both liquid and solid-like characteristics or viscoelasticity.
Agar
Agar is extracted from two major commercial sources of red seaweed: Gelidium sp. and Gracilaria sp. It consists of a mixture of agarose (gelling fraction) and agaropectin (non-gelling fraction), which is slightly branched and sulfated. In the commercial manufacture of food-grade agar, most of the agaropectin is removed during processing; hence, commercial agars are mainly composed of the agarose fraction.
Agarose is a linear polymer with a molecular weight of about 120,000, and is composed of a repeating dimer of d -galactosyl and 3,6-anhydro- l -galactosyl units connected via alternating a -(1-3) and b -(1-4) glycosidic linkages. Most applications for agar are based on its gelling ability and the fact that it is more stable to low pH and high-temperature conditions compared to other gelling systems. Gelation results from the formation of a network of agarose double helices, forming junction zones. These double helices are stabilized by the presence of water molecules bound inside the double-helical cavity. Agar solution gels at a temperature range of 90–103°C; so, when making an agar film, the solution and casting surface need to be maintained above the agarose gel-setting temperature to avoid premature gelation.
Agar film is clear and strong but is insoluble in water under ambient conditions, just like the parent agar. The linear, non-ionic nature of agar allows hydrated molecules to associate more intimately, forming a network that is stabilized by intensive intermolecular hydrogen bonding during the drying of the film.
Carrageenan
Carrageenan is a collective term for polysaccharides extracted from certain species of red seaweed of the family, Rhodophycae . Commercial sources are Eucheuma spinosum, Eucheuma cottonii, Gigartina spp. and Chondrus crispus. In terms of chemical make-up and structure, there are 4 types of carrageenan extracts: kappa I, kappa II, iota and lambda types. Different seaweeds produce different carrageenans, with one type being more predominant in any species of seaweed. A purer kappa and iota-carrageenan can be produced following potassium chloride precipitation, where kappa and iota fractions are made insoluble, and lambda is removed in the soluble phase. Physical separation of different species of seaweed, or picking of seaweed contaminants, is also a good way to obtain high-quality fractions.
Pectin
Pectin is commercially produced from the citrus peel as a by-product from extraction of lime, lemon and orange juices; or from apple pomace, the dried residue remaining after extraction of apple juice. Pectin is a heteropolysaccharide in its native state, but extraction with hot mineral acid removes most of the neutral sugars such as rhamnose, galactose, arabinose, etc., that comprise the branched or “hairy” regions of the polymer. Pectin is used in food mainly for its gelling property, which is influenced by its degree of esterification (DE). Gelation properties also translate into better interpolymer chain associations and film formation; therefore, pectins will generally form stronger films when gelation conditions (pH and/or presence of calcium) are met. Pectin films are generally not as strong as alginate films.
Chitosan
Chitosan is the principal derivative of chitin, the material comprising the exoskeletons of crustaceans and mollusks, and is produced by alkaline deacetylation of chitin. Chitosan is poly- b -(1-4)-2-amino-deoxy-d-glucopyranose. Typical commercial chitosan is about 85% deacetylated. In solution, chitosan forms micelle-like aggregates from fully acetylated segments of polysaccharide chains, interconnected by blocks of almost fully deacetylated polysaccharide, stretched by electrostatic repulsion. Chitosan forms strong films. When a plastic wrap made from chitosan was used for storage of fresh mangoes, the shelf life of the produce was extended up to 18 days, without any microbial growth and off-flavor.
Guar Gum
Guar gum is the ground endosperm of guar seed from the leguminous shrub Cyamopsis tetragonoloba, and is a galactomannan similar to fenugreek, tara and locust bean gum. It consists of a linear mannose chain linked by ß-(1-4) glycosidic bonds, with galactose substitution at the C-6 position of approximately every other mannose unit. Guar gum is a nonionic polymer, consists of long chain molecules (DP 10,000) than those found in locust bean gum.
Xanthan Gum
Xanthan gum is a polysaccharide that is produced by the bacterium, Xanthomonas campestris, during a fermentation process involving a carbohydrate substrate and other growth-supporting nutrients. This gum is an excretion to protect bacterial cells when the pH of the fermentation medium becomes too low and unfavorable for growth. It is likely because of this native protective function that xanthan gum demonstrates superior stability to acid over other gums. It is stable over a wide range of pH, from 1 to 13; very resistant to enzyme hydrolysis; tolerant of high salt, high sugar, and high alcohol systems; stable at boiling temperatures; and tolerates retort processing better than most other gums.
Xanthan solution is highly pseudoplastic and shows a drastic decrease in viscosity with increasing shear rate. It can have a soft, gel-like consistency, depending on concentration and shear applied, typical of a pseudoplastic fluid. The superior suspending property of xanthan gum is attributed to its weak-gel, shear-thinning properties. It hydrates rapidly in cold water, even in the presence of high levels of sugar, salt or alcohol. The consistent water-holding ability of xanthan may be used for control of syneresis and to retard ice recrystallization in freeze-thaw situations.
Polysaccharide films
Polysaccharide films have poor moisture barriers but have good mechanical properties. Polysaccharides that have been used for film-forming are cellulose and cellulose derivatives, starch, some hydrocolloids like carrageenan, pectin, etc. (Rico and Tonnes, 1990). Lazarus et al. (1976) observed that polysaccharide coating increase moisture, and reduce the perception of warmth over flavour induced by oxidation. Treatment with chitosan also induces the activity of chitinase enzymes which are made by the plant for self-protection against pathogens.
Protein films
Different proteinaceous substances that have been used for film-forming are milk proteins, i.e. casein and whey protein, wheat gluten, corn protein, soy protein, etc. The use of casein and whey protein for film-forming is very recent. Although protein film is a poor moisture barrier due to their hydrophilic nature they have considerable mechanical and gas barrier properties. Whey protein films and coatings are excellent aroma and oxygen barrier, moisture barrier as well as gloss enhancers (McHugh and Krochta, 1994; Lee et al., 2002). Avena et al. (1994) studied one of the oldest known edible coatings used for packing meat products (made from the collagen) casein proteins, derived from milk, which have been used in emulsion-based coatings to reduce water loss in zucchini.
Whey Protein Isolate/plasticiser formulation of the edible coating was found to be effective in high sugar products like confectioneries as a source of glaze in the confectionery industry (Lee et al., 2002). Whey protein films and coatings are excellent aroma, oxygen, and moisture barriers as well as gloss enhancers (Mc.Hugh and Krotcha 1994, Lee et al., 2002). Whey protein-based films have an extra edge over many other biopolymer films because of excellent nutritional value, bland flavour, and capability to carry flavourings and functional ingredients. Properties of lipid and polysaccharide-based edible coatings have been reviewed by Kester and Fennema (1986), Guilbert (1986). According to Banerjee and Chen (1995), WPC films have a good water vapour barrier and mechanical properties that were comparable with those of films from other commercial milk proteins.
Edible films made from whey protein isolate (WPI) and plasticized with sucrose are excellent oxygen barriers that are flexible, tough and highly glossy (Dangran and Krochta, 2006). Moreover, WPC is more environment-friendly because it degrades faster than WPI films (Li and Chen, 2000). These characteristics are well desirable for coating applications. Gennadios et al. (1990) noticed that zein protein has also been used effectively as coatings for confectionery products. Fruit and vegetable products, however, have not been coated successfully with protein-based coating, primarily since these types of coatings do not provide effective moisture barriers. Corn zein protein has been used to prevent oxidative rancidity, staling, and sogginess in nut products.
Lipid film
Lipid is very hydrophobic and therefore, acts as a good moisture barrier. However, their mechanical properties are inferior to protein and polysaccharide based films. Lipids like bees wax, carnauba wax acetylated monoglycerides, etc. have been used for film making (Banarjee and Chen, 1995). Fatty acids and monoglycerides are used in coatings mainly as emulsifiers and dispersing agents. Fatty acids are generally extracted from vegetable oils, while monoglycerides are prepared by transesterification of glycerol and triglyceride.
Composite films and bilayer coatings
The composite film consists of two or more components so that the characteristics of the film are enhanced by the individual contribution from each component. Therefore, protein-lipid or polysaccharide–lipid composite films are more effective than pure protein or polysaccharide films (Kester and Fennema, 1986). Baldwin (1991) noticed that composite and bilayer coatings are the edible coatings of the future. These two types of coatings work to combine the beneficial properties of coating ingredients to create a superior film. Composite films of the future may consist of hydrophilic particles within a hydrophilic matrix.
Such a configuration could give a water-soluble coating with good water vapor barrier properties. Wong et al. (1994) studied that bilayer coatings, which already have been used to a limited extent, combine the water barrier properties of lipid coatings with the greaseless feel and good gas permeability characteristics of polysaccharide coatings. Such a bilayer coating has been shown to reduce gas exchange, and result in higher internal carbon dioxide and lower oxygen concentrations in cut apple pieces. Unfortunately, little or no data exists showing the effects of bilayer coatings on whole fruits or vegetables and other food materials.
‘Processed Food Industry’: The Voice of Food Processing Industry
Processed Food Industry (PFI) is a premier English-language monthly B2B publication (ISSN 09721649) headquartered in New Delhi, catering to the vibrant and ever-evolving food processing industry. While we don’t claim to be the largest or most widely read, our proud legacy of over 27 years—publishing continuously since 1997—has earned us the trust of industry professionals as a reliable source of insights and information.
If your goal is to tap into the booming Indian and South Asian markets to promote your equipment, technology, software, or consumables, PFI is your strategic partner. With our hybrid approach across print, web, and social media, we help you establish strong brand recognition rooted in market relevance. Backed by a team of top-tier technical writers, we’re ready to work closely with you and your customers to craft compelling content that drives results.
India and South Asia’s food industry is expanding rapidly, driven by efficiency and cutting-edge innovations. Don’t miss the opportunity to elevate your brand and engage with this dynamic market. Get our 2025 media kit to fine-tune your marketing strategy, increase your visibility, and convert potential customers into valuable conversations. Additionally, ask for a sample copy of our monthly magazine and experience the quality and relevance we deliver.
Let us help you define your role in the future of the food processing industry.

Have a news or topic to share with industry? Write to us [email protected]
Trackbacks/Pingbacks